Magnetic Resonance Imaging
Although X-rays do an excellent job of imaging bones, they aren't as good for imaging tissue. A better technique for studying tissue is magnetic resonance imaging (MRI). This technique locates hydrogen atoms by interacting with their magnetic nuclei. Since hydrogen atoms are common in both water and organic molecules, finding hydrogen atoms is a good way to study biological tissue.
The nucleus of an ordinary hydrogen atom, 1 H, is a proton. Protons, like electrons, have two possible internal quantum states, usually called spin-up and spin-down. Calling it spin is appropriate because spin-up and spin-down protons have equal but oppositely directed angular momentum. When electric charge and rotation are both present, it's not surprising that magnetism is, too; electric currents are magnetic, after all. Sure enough, protons have magnetic dipoles—equal north and south poles at a distance from one another.
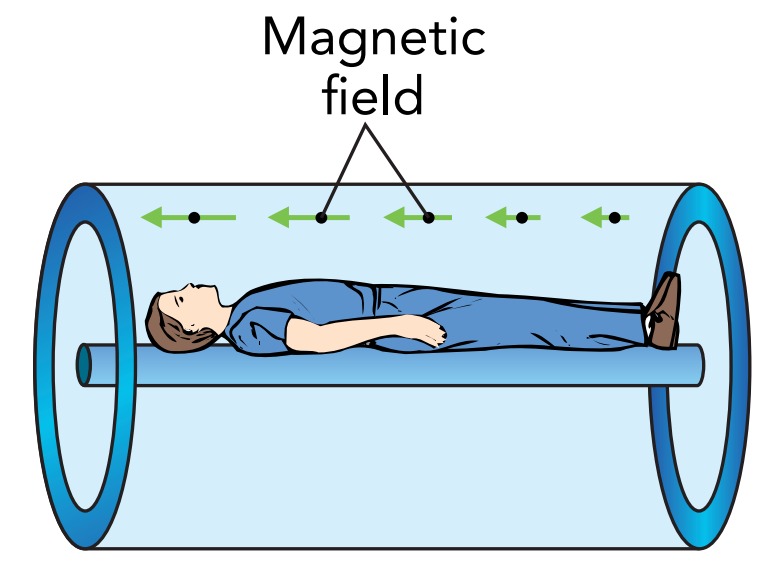
A spin-up proton acts as though it has its north pole on top, while a spin-down proton acts as though it has its south pole on top.
When a proton is immersed in a magnetic field, it tends to align its magnetic dipole with that field. Doing so minimizes its magnetic potential energy. But although protons would align perfectly with the field at absolute zero, they are less successful near room temperature. Thermal energy agitates the protons so that, even in a strong, upward-pointing magnetic field, spin-up protons only slightly outnumber spin-down protons.
In that upward-pointing magnetic field, each proton has two possible quantum states: alignment with the field (spin-up) or anti-alignment (spin-down). Because alignment reduces the proton's magnetic potential energy, it's the ground state—the lower energy of the two possible states. The anti-aligned state is the excited state. With its two possible states, ground and excited, a proton in a magnetic field can exhibit many of the behaviors when looking at atoms. Most important, the proton can experience radiative transitions between its two states. A groundstate proton can absorb a photon while making a radiative transition to its excited state, and an excited-state proton can emit a photon while transiting to its ground state.
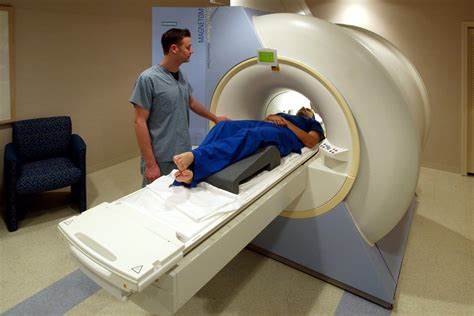
Atom can absorb or emit only certain photons, photons carrying exactly the right amount of energy to shift the atom from one quantum state to another. For example, neon signs are red because neon atoms have states that are separated in energy by the energy of red photons. Similarly, a proton in a magnetic field can absorb or emit only certain photons, photons carrying exactly the right amount of energy to shift the proton from one quantum state to the other. However, unlike a neon atom, which always interacts with red photons, a proton in a magnetic field interacts with photons that vary in “color” according to the strength of the magnetic field. That's because the energy separating the proton's two states is proportional to the magnetic field in which it resides. As a result, the photon energy needed to cause radiative transitions between the proton's two states is also proportional to the magnetic field. If the field changes, so does the photon energy.
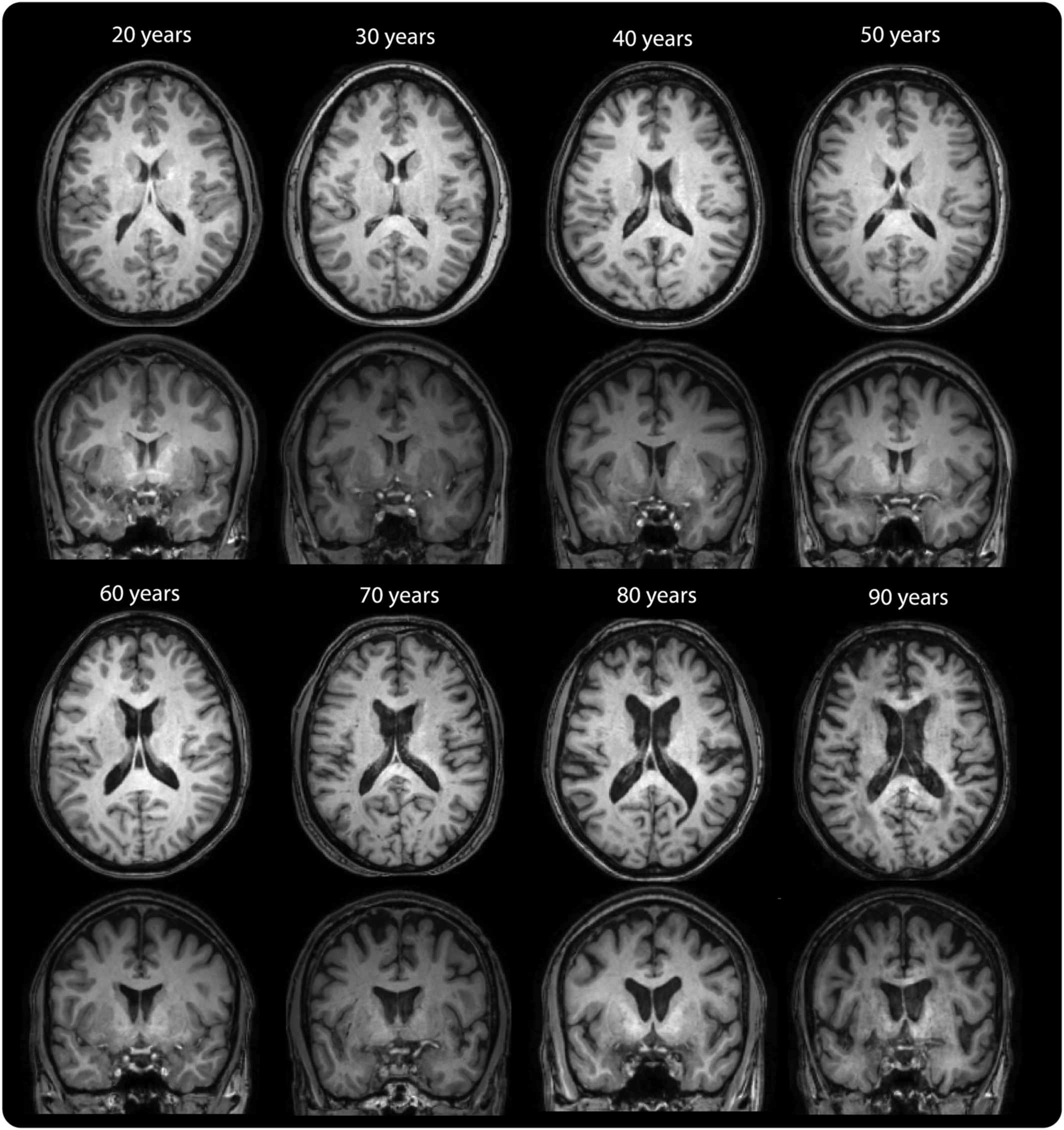
When a patient enters the strong magnetic field of an MRI machine, the protons in the patient's body respond to the field and a small excess of aligned protons develops. Only these excess aligned protons matter to the MRI machine because effects due to the remaining protons, which are equally aligned and anti-aligned, cancel completely. The excess aligned protons are in their ground state, and they are what the MRI machine studies.
The MRI machine interacts with these ground-state protons using radio wave photons, photons with energies equal to the energy difference between their ground and excited states. The protons can absorb and subsequently emit those radio wave photons, and they can also exhibit a variety of fascinating and useful quantum interference effects.
If the protons in the patient's body were all experiencing exactly the same magnetic field, they would all interact with the same radio wave photons—but the protons don't all experience the same field. The MRI machine introduces a slight spatial variation to its magnetic field. Because the magnetic field is different for different protons, only some of them can interact with radio wave photons of a particular energy. This selective interaction is how the MRI imager locates protons within a patient.
In its simplest form, an MRI machine applies a spatially varying magnetic field to the patient's body. It then sends various radio waves through the patient and looks for the radio wave photons to interact with protons. Since only a proton that is experiencing the right magnetic field can interact with a particular radio wave photon, the MRI machine can determine where each proton is by the photons with which it interacts. By changing the spatial variations in the magnetic field and adjusting the energies of the radio wave photons, the MRI machine gradually locates the protons in the patient's body. It builds a detailed three-dimensional map of the hydrogen atoms. A computer manages this map and can display cross-sectional images of the patient from any angle or position.