Light
Light consists of very-high-frequency, very-short-wavelength electromagnetic waves. Light's frequencies are so high that normal antennas can't handle it. Instead, it's absorbed and emitted by the individual charged particles in atoms, molecules, and materials. Because of its special relationship with the charged particles in matter, light is important to physics, chemistry, and materials science. Moreover, it's one of the principal ways by which we interact with the world around us.
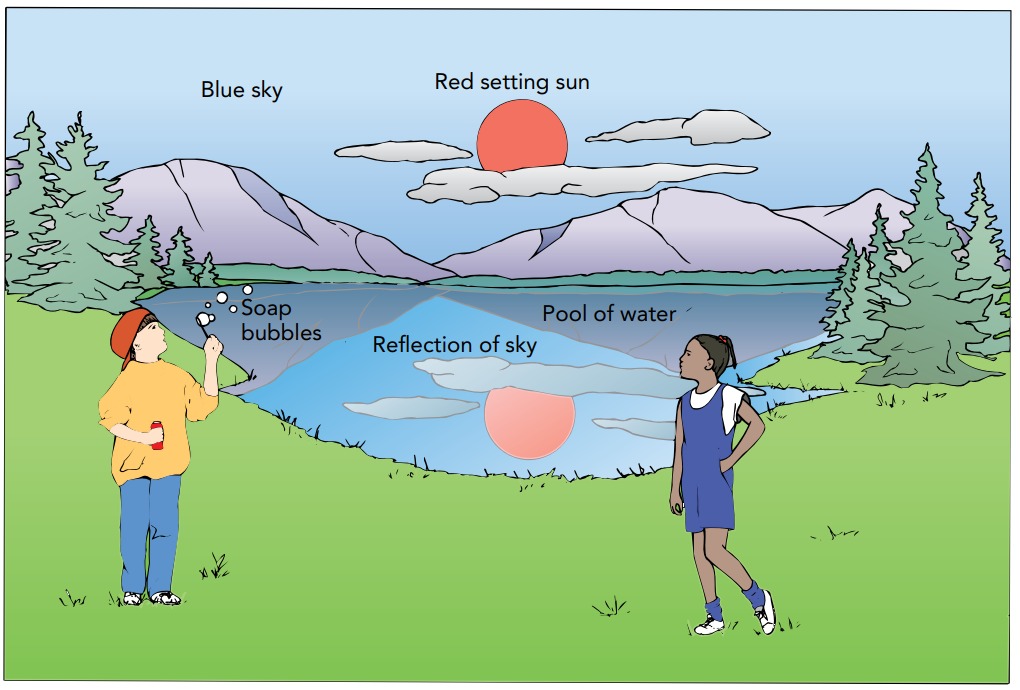
For thousands of years, people have marked the passage of time by the rising and setting of the sun over the horizon. The sun first appears as a red disk in the east every morning, rises white in the blue sky, and then sets once again as a red disk in the west. The sunlight that we see takes about 8 minutes to travel the 150 million km or 93 million miles from the sun to our eyes and provides most of the energy and heat that make life on Earth possible. Although the light in sunlight is really just another electromagnetic wave, it's so important to everyday life that it deserves special attention. Therefore we'll begin by looking at how sunlight interacts with our world.
Electromagnetic waves can have any wavelength, from thousands of kilometers to a fraction of the width of an atomic nucleus. The radio waves and microwaves that have wavelengths longer than 1 mm. we turn our attention to shorter-wavelength radiation. In particular, we'll study electromagnetic waves with wavelengths between 400 nanometer and 750 nanometer. These are the electromagnetic waves that we perceive as visible light and the principal components of sunlight.
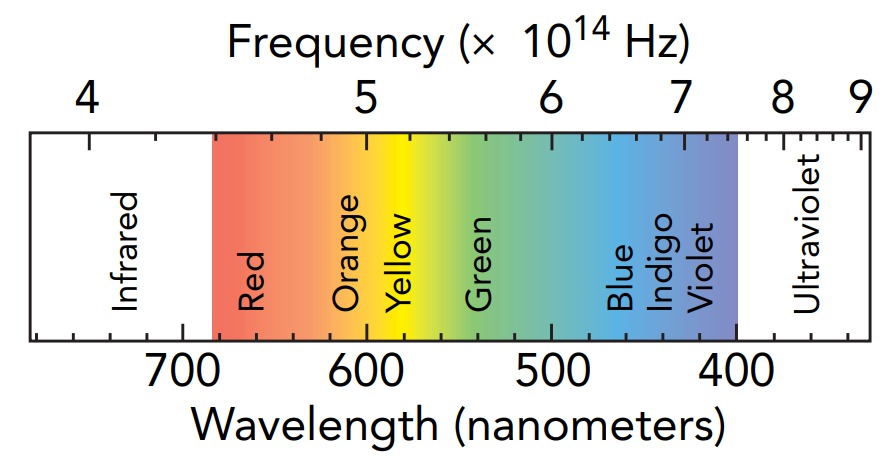
Because the electromagnetic waves in sunlight have such short wavelengths, their frequencies lie between 10 raised to 14 and 10 raised to 15 Hz. As one of these waves of sunlight passes by, its electric field fluctuates back and forth almost 1 million billion times each second. Since producing microwaves, which have much longer wavelengths and much lower frequencies, already requires specialized components and tiny antennas, what can possibly emit or absorb light waves? The answer is the individual charged particles in atoms, molecules, and materials. These tiny particles can move extremely rapidly, often vibrating about at frequencies of 10 raised to 14 Hz, 10 raised to 15 Hz, or even higher. As these charged particles accelerate back and forth, they emit light waves. Similarly, passing light waves cause individual charged particles in atoms, molecules, and materials to accelerate back and forth, thereby absorbing the light waves as well.
Sunlight
Sunlight originates at the outer surface of the sun, in a region called the photosphere. There, atoms and other tiny charged systems (mostly atomic ions and electrons) jostle about at 5800 K. Since these charged particles accelerate as they bounce around, they emit electromagnetic waves.
Because the sun's surface emits light through the random, thermal motions of its charged particles, the distribution of wavelengths it emits is determined only by its temperature. It emits a blackbody spectrum. Because the photosphere's temperature is 5800 K, the jostling motions are extremely rapid and most of the sunlight falls in the visible portion of the electromagnetic spectrum.
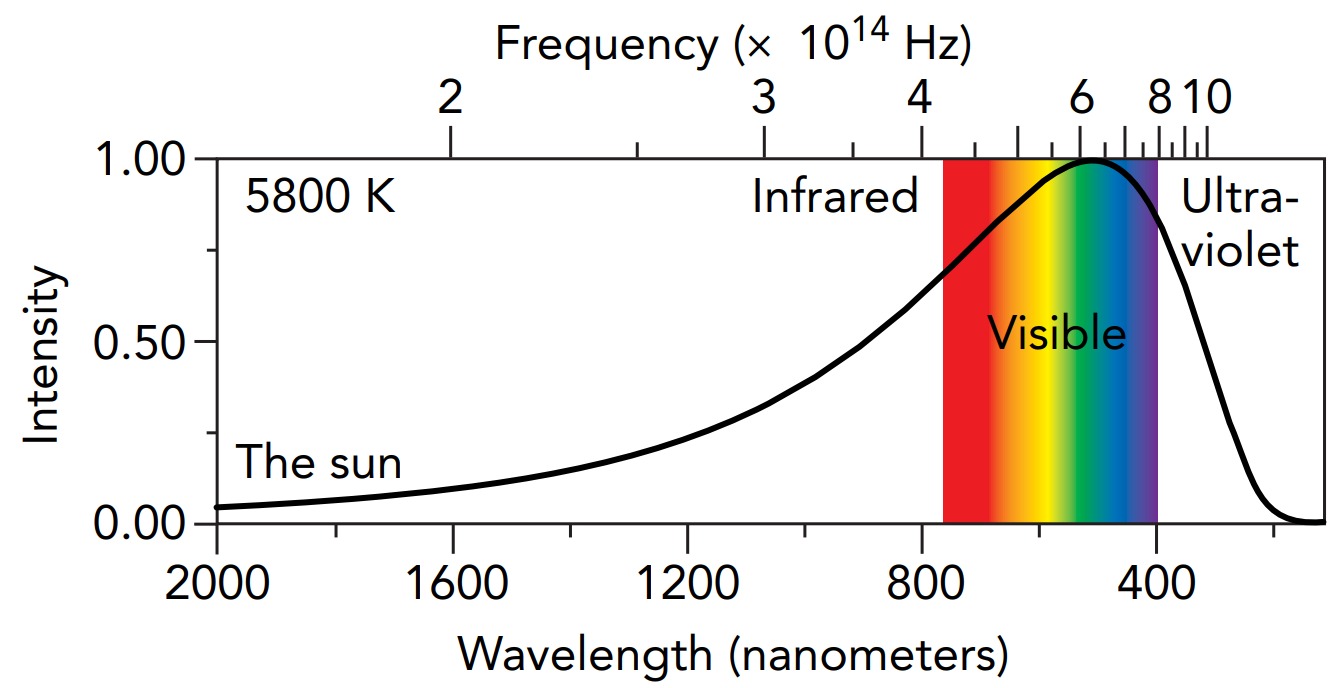
However, not all sunlight is visible. On the long-wavelength, low-frequency side of visible light is infrared light. We can't see infrared light with our eyes, but we feel it when we stand in front of a hot object. In sunlight, infrared light is produced by charges that are accelerating back and forth more slowly than average.
On the short-wavelength, high-frequency side of visible light is ultraviolet light. We can't see ultraviolet light either, but we're aware of its presence because it induces chemical damage in molecules. It causes sunburns and encourages skin to tan. In sunlight, ultraviolet light is produced by charges that are accelerating back and forth more rapidly than average. In short, Sunlight comes from the sun's photosphere, where the temperature is 5800 K. This light has a blackbody distribution of wavelengths, with much of its intensity concentrated in the visible portion of the overall electromagnetic spectrum. The visible portion of the spectrum of sunlight. Each wavelength of visible light has a particular frequency and is associated with a particular color. At the ends of the visible spectrum are invisible infrared and ultraviolet lights.
Sunlight travels from the sun to Earth at the speed of light. It's one of the fundamental constants of nature, with a defined value of 299,792,458 meter per second in empty space. The speed of light is set by the relationships between the electric and magnetic fields. Let's look at what happens to it when it enters a region that's not empty. Sunlight eventually reaches Earth's atmosphere and, when it does, several interesting things happen.
First, the sunlight slows down as its electric and magnetic fields begin to interact with the electric charges and magnetic poles in the atmosphere. Light polarizes the molecules it encounters-its electric field displaces positive charges from negative charges, and its magnetic field displaces north poles from south poles. These polarization effects delay light's passage so that it travels more slowly. Since most transparent materials respond much more strongly to light's electric field than to its magnetic field, we'll concentrate on only electric effects.
The factor by which light slows down in a material is known as the material's index of refraction. Light travels particularly slowly through materials that are easy to polarize, and some of them have indices of refraction of 2 or even 3. Because air near sea level is only slightly polarizable, however, its index of refraction is just 1.0003. Although this reduction in light's speed is too small to notice directly, we do notice the polarized air particles that cause it. These polarized air particles are what makes the sky blue.
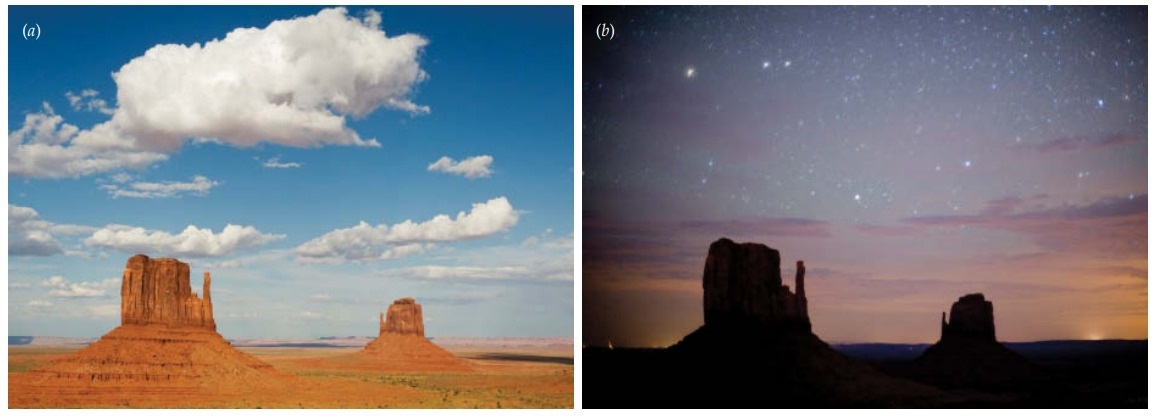
The particles in air consist of individual atoms and molecules, small collections of atoms and molecules, water droplets, and dust. As a wave of sunlight passes through one of these particles, the particle becomes polarized. Its electric charges accelerate back and forth as the sunlight's electric field pushes them around, and they reemit a new electromagnetic wave of their own.
This new wave draws its energy from the original wave. In effect, the particle acts as a tiny antenna, temporarily receiving part of the electromagnetic wave and immediately retransmitting it in a new direction. This process, whereby a tiny particle redirects the path of a passing light wave, is called Rayleigh scattering, after the English physicist Lord Rayleigh (John William Strutt, 1842–1919).
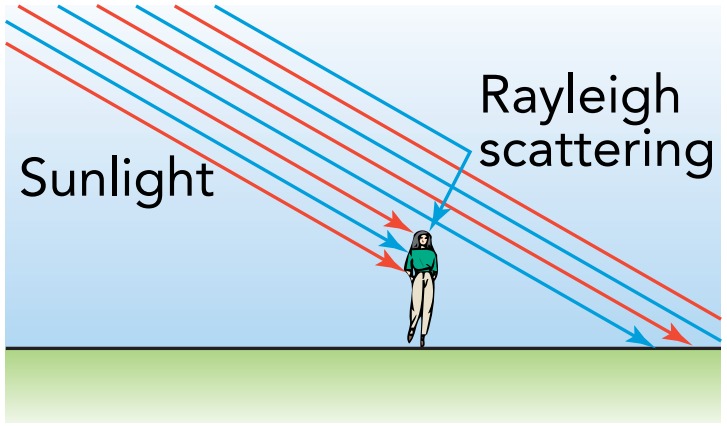
Although most sunlight travels directly to our eyes, some of it undergoes Rayleigh scattering and reaches us by more complicated paths. We see the direct light as coming from the brilliant disk of the sun, but the scattered light gives the entire sky a fairly uniform blue glow. Why is this glow blue?
The sky's blue color comes about because the tiny air particles that Rayleigh-scatter sunlight are too small to make good antennas for that light. A dipole antenna works best when it is about half as long as the wavelength of its electromagnetic wave. The air particles make particularly bad antennas for long-wavelength red light, so very little red sunlight undergoes Rayleigh scattering on its way through the atmosphere. However, the air particles make less poor antennas for short-wavelength blue light. Some of the violet, indigo, and blue sunlight does Rayleigh-scatter and reaches our eyes from all directions. We see this Rayleigh-scattered light as the bluish glow of the sky.
Rayleigh scattering not only makes the sky blue; it also makes the sunrises and sunsets red. As the sun rises or sets, its light must travel long distances through Earth's atmosphere to reach your eyes. Its path is so long that most of the violet, indigo, and blue light Rayleighscatters away miles to your east or west and all you see is the remaining red light. Sometimes the whole local sky appears reddish because there simply isn't any bluish light left to scatter toward you. Sunrises and sunsets are particularly colorful when extra dust or ash is present in the atmosphere to enhance the Rayleigh scattering. Air pollution, forest fires, and volcanic eruptions tend to create unusually red sunrises and sunsets.
In contrast, clouds and fog appear white because they're composed of relatively large water droplets. These droplets are larger than the wavelengths of visible light and scatter all of sunlight's wavelengths equally well. Although this scattering is often so effective that you can't see the sun's disk through a cloud, it doesn't give the cloud any color. The cloud simply looks white.
Sometimes water droplets do separate the colors of sunlight. When sunlight shines on clear round raindrops as they fall during a storm, these raindrops can create a rainbow. To understand how clear spheres of water can bend sunlight's path and separate it according to wavelength, we must understand three important optical effects: refraction, reflection, and dispersion.
Let's begin by looking at what happens when a wave of sunlight passes directly through a raindrop. Because water is more polarizable than air, the wave slows down inside the raindrop and its cycles bunch together. This bunching effect reduces the light's wavelength inside the drop. Light's frequency remains unchanged because the cycles can't disappear, but they move more slowly.
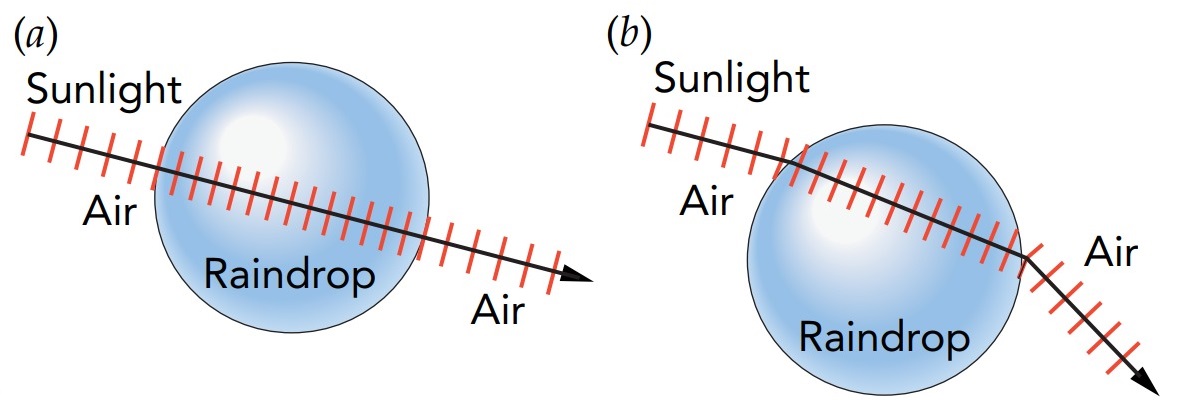
If a narrow wave of sunlight is aimed directly through the center of the raindrop, it will follow a straight path and emerge essentially unaffected from the other side. However, if that wave strikes the raindrop near the top, it will bend as it enters the water. This occurs because the lower edge of the wave will reach the water first and slow down, the upper edge will then overtake it and the wave will bend downward. The wave will head more directly into the water.
As the wave leaves the raindrop, its upper edge emerges first and speeds up while the lower edge lags behind. The wave bends downward even further and heads less directly into the air and away from the water.
This bending of sunlight at the boundaries between materials is refraction. It occurs whenever sunlight changes speeds as it passes through a boundary at an angle. If sunlight slows down at a boundary, it bends toward the line perpendicular to the boundary and heads more directly into the new material. If sunlight speeds up at a boundary, it bends away from the line perpendicular to the boundary and heads less directly into the new material. The amount of the bend increases as the speed change increases.
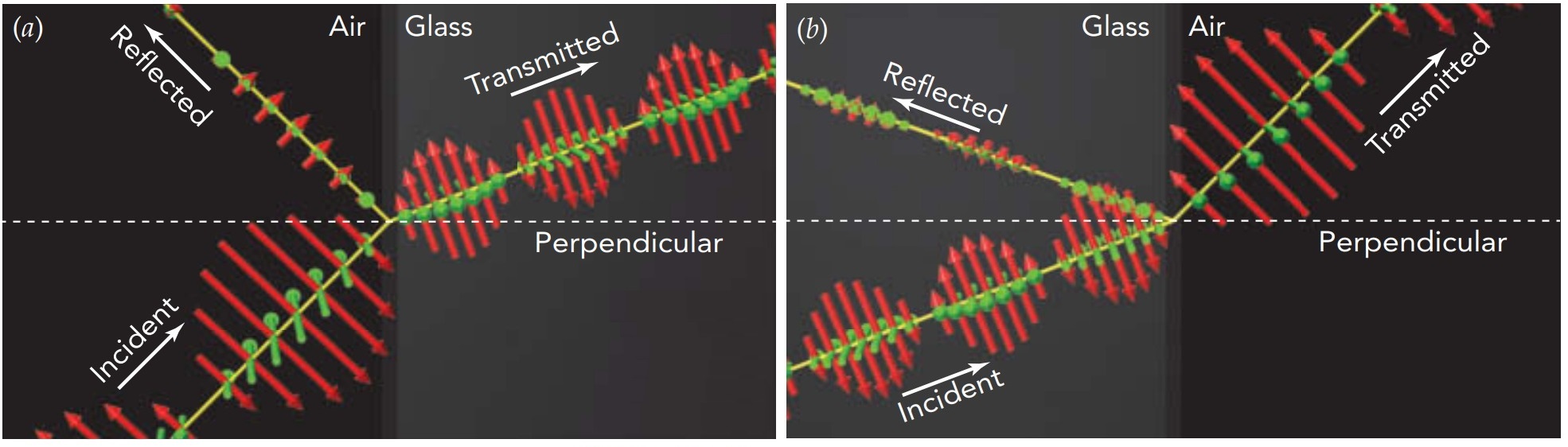
However, part of the sunlight striking a boundary doesn't pass through the boundary at all. Instead, it reflects. Wave reflection specifically results in the changes in wave speed at a boundary. However, the more general cause of wave reflections is an impedance mismatch, an abrupt change in how the wave moves through its environment. In general, impedance measures a system's opposition to the passage of a current or a wave. For an electric current, impedance measures how much voltage is needed to propel a given current. For an electromagnetic wave, impedance measures how much electric field is needed to produce a given magnetic field. Impedance effects are common in nature and also apply to mechanical waves and mechanical currents. When sound and water waves encounter impedance mismatches, they partly reflect as echos.
The impedance of empty space is high because there an electric field has nothing to aid it in producing a magnetic field. Inside most materials, however, the electric field has help. The electric field polarizes the material, which then helps to create the magnetic field. Because of this assistance, the impedance of most materials is much less than that of empty space. Since air is almost empty space, the boundary between air and water is an impedance mismatch for light.
Passing through an impedance mismatch upsets the balance between a light wave's electric and magnetic fields. To compensate for this imbalance, part of the incoming wave reflects off the boundary. Thus some sunlight reflects each time it enters or leaves a water droplet. The fraction of light that reflects depends on the severity of the impedance mismatch, but it is typically 4% between air and most transparent materials, including water. In contrast, metals polarize so easily that their impedances are essentially zero and they reflect light almost perfectly.
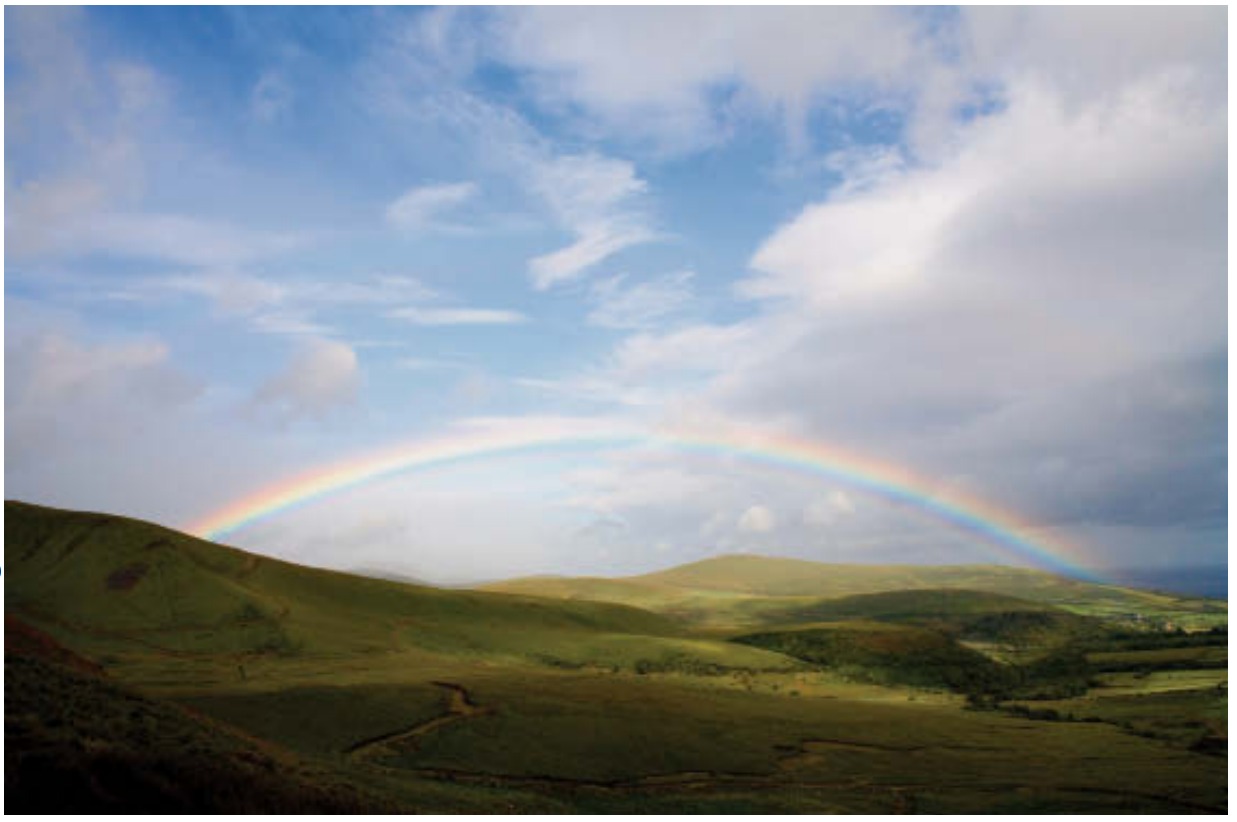
There is one more important point about sunlight's passage through water: red light travels about 1% faster through water than violet light does. That's because higher-frequency violet light polarizes the water molecules a little more easily than lower-frequency red light does and that increased polarization slows down the violet light. This frequency dependence of light's speed in a material is dispersion. Dispersion affects refraction. The more light slows as it enters a raindrop, the more it bends at the boundary. Since violet light slows more than red light, violet light also bends more and the different colors of sunlight follow somewhat different paths through the raindrop.
A rainbow is created when raindrops separate sunlight according to color. To see the rainbow, you stand with the sun at your back and look up at the sky. When sunlight hits the raindrops, they redirect some of that light back toward you. Since each raindrop redirects light only in a narrow range of angles, you can't see light from every raindrop. Only the raindrops in a narrow arc of the sky redirect visible light toward you. This arc appears brightly colored because raindrops at the inner edge of the arc send violet light toward you while raindrops at the outer edge of the arc send red light toward you. In between, you see all the colors of the rainbow.
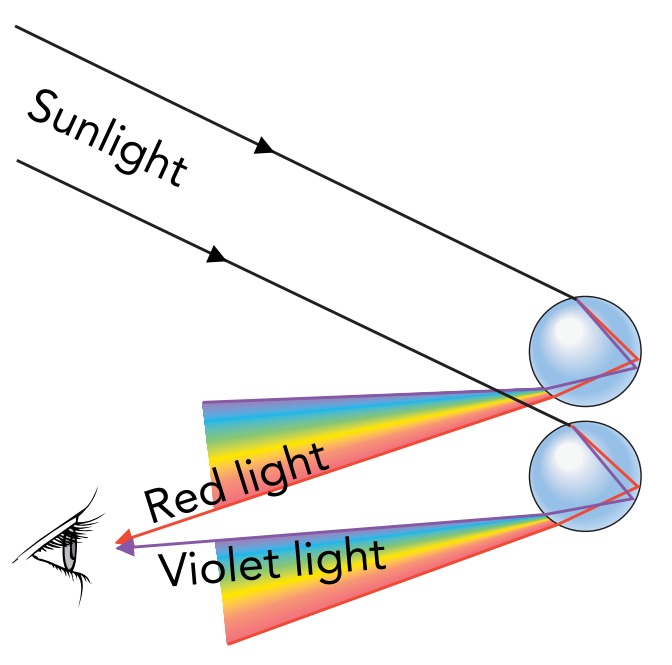
When the light inside the raindrop strikes the back surface, most of it leaves the drop and is lost. A small fraction of the light reflects from that surface, however, and continues to travel through the raindrop. When this light reaches the raindrop's front surface, most of it leaves the drop. Violet light bends more strongly than red light as they reenter the air, so the different colors of light leave the drop heading in different directions. Since violet light is redirected more upward than red light, you see violet light coming toward you from the lower raindrops. Red light is redirected more downward so you see it coming toward you from the upper raindrops. Thus the upper arc of the rainbow is red, while the lower arc is violet.
Sunlight and Polarizing Sunglasses
All sunglasses absorb some of the sunlight passing through them, but the best ones absorb horizontally polarized light much more strongly than vertically polarized light. These polarizing sunglasses dramatically reduce glare by eliminating most of the light reflected from horizontal surfaces.
When light strikes a transparent surface at right angles, about 4% of that light is reflected, regardless of its polarization. When light strikes a horizontal surface at a shallow angle, however, its polarization profoundly affects its reflection. A light wave that has a horizontal electric field (essentially horizontally polarized light) reflects strongly from the surface. In contrast, a light wave that has a horizontal magnetic field (essentially vertically polarized light) barely reflects at all.
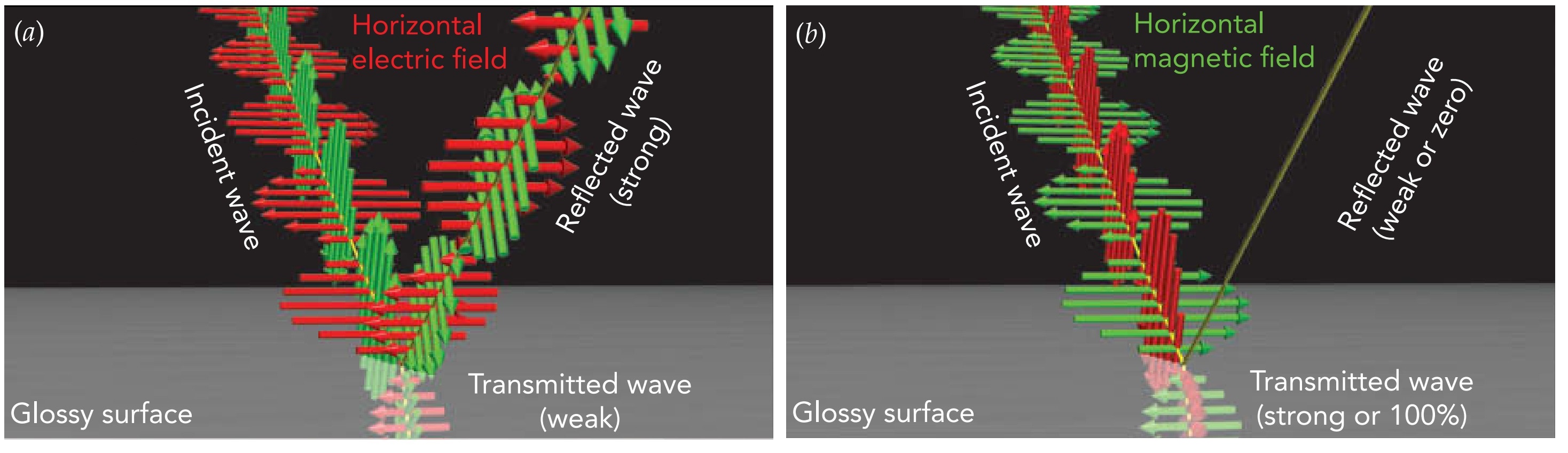
When the wave's electric field is horizontal, it pushes electric charges back and forth along a horizontal surface. Charges shift relatively easily in that direction, so the surface polarizes well and reflects the wave strongly. The shallower the angle between the surface and the incident light wave, the larger the surface's polarization and the greater the reflection. Horizontally polarized light thus reflects strongly from horizontal surfaces and its reflection increases as its angle to the surface becomes shallower.
When the wave's electric field is vertical or nearly vertical, it pushes electric charges in and out of a horizontal surface. Since the charges can't leave the surface, the surface polarizes ineffectually and reflects the wave weakly. The polarization and reflection become even weaker as the angle becomes shallower until, at Brewster's angle, the wave doesn't reflect at all. Vertically polarized light thus reflects weakly from horizontal surfaces and that reflection drops to zero at Brewster's angle. For angles shallower than Brewster's angle, however, the reflection becomes stronger as the angle becomes shallower.
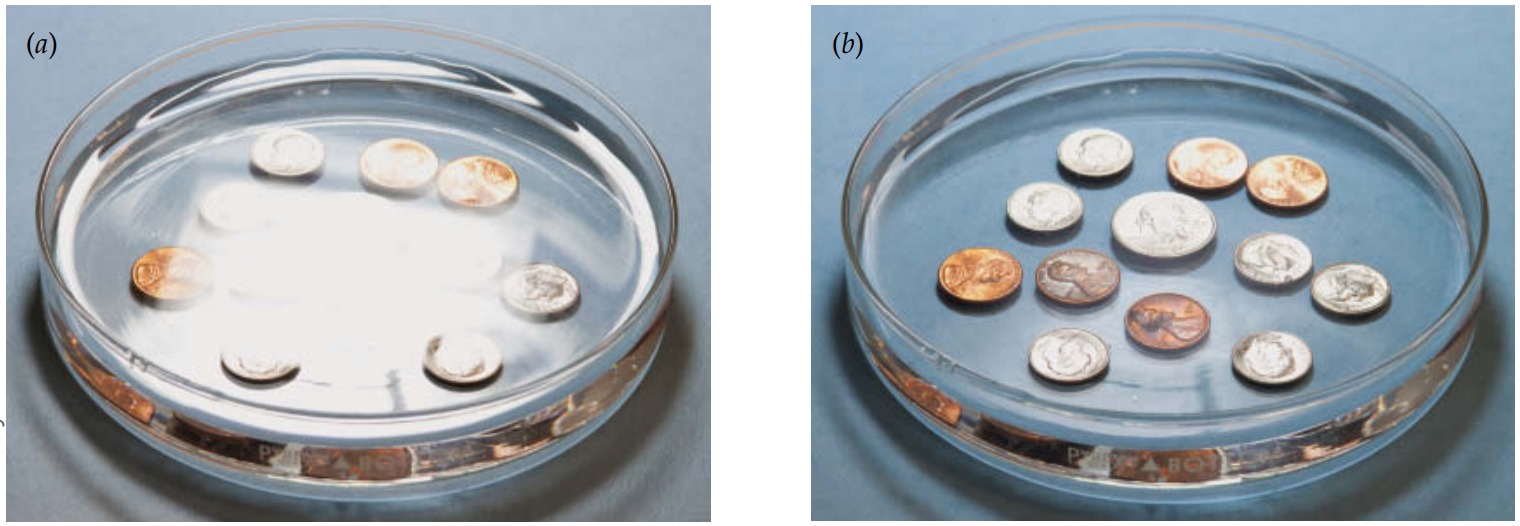
Although direct sunlight is an even mixture of vertically and horizontally polarized waves, the shallow-angle glare reflecting from the horizontal surfaces of water, pavement, or painted car hoods is mostly horizontally polarized waves. Polarizing sunglasses are designed to absorb horizontally polarized light, which is why they are so effective at reducing glare.