Laser Shows
Since its discovery in late 1950s, lasers have found countless uses, from cutting metal and clearing human arteries to surveying land and playing CDs and DVDs. However, lasers are more than just novel applications of old ideas. Instead, they bring together quantum and optical physics to produce a new type of light. This light is radically different from the light produced by incandescent and fluorescent lamps, and its properties make it particularly useful for many applications.
Laser and Laser Light
To understand lasers, you must understand how laser light differs from the normal light emitted by hot objects or by individual atoms in an electrical discharge. Each particle of normal light, each photon, is emitted willy-nilly without any relationship to the other light particles being emitted nearby. Because of this light's independent and unpredictable character, it's called spontaneous light and its creation is called spontaneous emission of radiation.
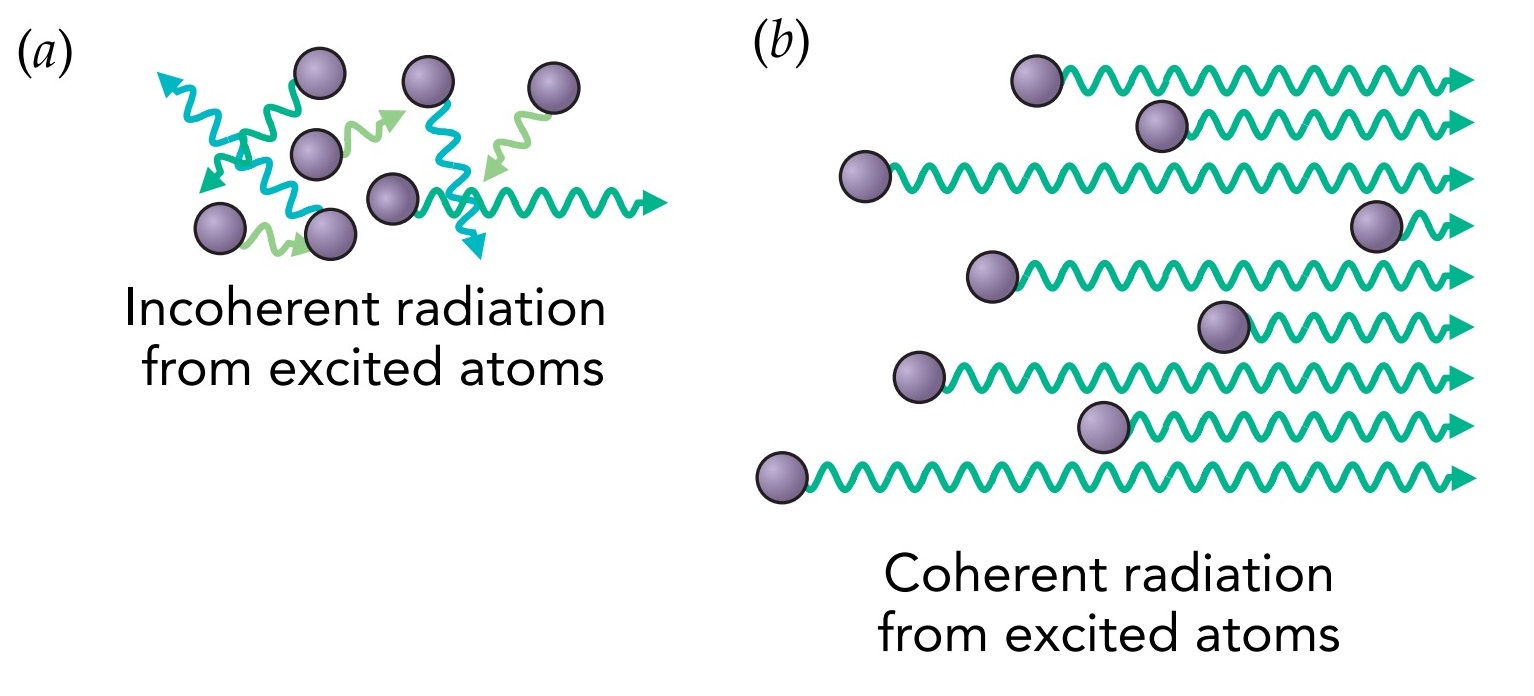
Theoretical work by Albert Einstein and others in the 1920s and 1930s predicted the existence of a second type of light, stimulated light, that can be created when an excited atom or atomlike system duplicates a passing photon. Although this stimulated emission of radiation can occur only when the excited atom is capable of emitting the duplicate photon spontaneously, the copy that it produces is so perfect that the two photons are absolutely indistinguishable. Together, these two photons form a single electromagnetic wave.
To get a slightly better picture of how such stimulation occurs, think about an isolated atom in an excited state. That atom will eventually return to its ground state, but it must emit one or more photons for this to happen. That atom waits around in the excited state until it begins a spontaneous radiative transition. During the transition, one of the atom's electrons accelerates back and forth, and the atom emits a photon.
If a similar photon passes through the atom as it's waiting in the excited state, that photon's electric field can stimulate the radiative transition process through sympathetic vibration. The field pushes and pulls on the atom's electrons and makes them accelerate back and forth. Although this effect is small, it may be enough to trigger the emission of light. If the atom does emit light, the photon it produces will be a perfect copy of the stimulating photon.
When this stimulated emission process was first discovered, people immediately recognized that it made light amplification possible. If enough excited systems could be assembled together, a single passing photon could be duplicated exactly over and over again. Instead of a single particle of light, you would soon have thousands, or millions, or even trillions of identical light particles.
Implementing this idea had to wait until the late 1950s, however, when the technical details of how to actually achieve light amplification were worked out. In 1960, the first laser oscillators were constructed. These were devices that emitted intense beams of light in which each particle of light was identical to every other particle of light. A single particle of light had been duplicated by the stimulation process into countless copies.
When individual excited atoms or atomlike systems emit light through spontaneous emission, the particles of light head off separately as many independent electromagnetic waves. Light consisting of many independent electromagnetic waves is called incoherent light.
However, when that same collection of excited atoms or atomlike systems emit light by stimulated emission, all of the light particles are absolutely identical and form a single electromagnetic wave. Unlike electrons, which are Fermi particles, many identical photons can have the same quantum wave because photons are Bose particles and Bose particles don't obey the Pauli exclusion principle. Light consisting of many identical photons and a single electromagnetic wave is called coherent light. Because of its singlewave nature, coherent light exhibits remarkable interference effects. These effects are easily seen in the coherent light emitted by lasers.
Light Amplifiers
Producing coherent light requires amplification. You must start with only one particle of light and duplicate it many times. The basic tool for this duplication of light is a laser amplifier. When weak light enters an appropriate collection of excited atoms or atomlike systems-the laser medium-that light is amplified and becomes brighter. The new light has exactly the same characteristics as the original light, but it contains more photons.
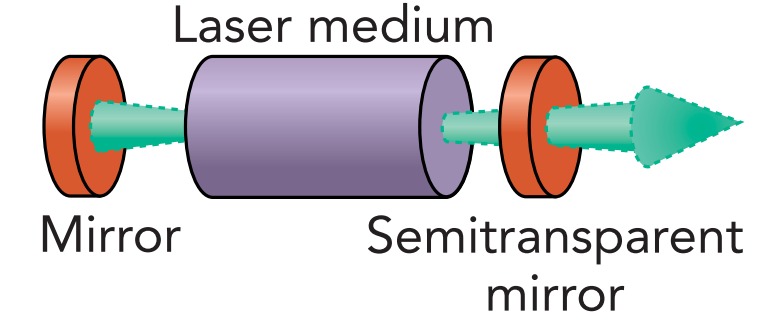
When we think of lasers, however, we rarely imagine a device that duplicates photons from somewhere else. We usually picture one that creates light entirely on its own. To do that, the laser must produce the initial particle of light that it then duplicates to produce others. A laser oscillator is a device that uses the laser medium itself to provide the seed photon, which it then duplicates many times. If a laser medium is enclosed in a pair of carefully designed mirrors, it's possible for the stimulation process to become self-initiating and self-sustaining. However, the mirrors must be curved properly and must have the correct reflectivities. One mirror must normally be extremely reflective, while the other must transmit a small fraction of the light that strikes its surface.
When the laser medium is placed between the two mirrors, there is a chance that a photon, emitted spontaneously by one of the excited systems, will bounce off a mirror and return toward the laser medium. As that returning photon passes through the laser medium, it's amplified. Because the photon was emitted by one of the excited systems, it has the right wavelength to be amplified by other excited systems.
By the time the original photon leaves the laser medium, it has already been duplicated many times. This group of identical photons then bounces off the second mirror and returns for another pass through the laser medium. It continues to bounce back and forth between the mirrors until the number of identical photons in the collection is astronomical.
Eventually there are so many identical photons that the laser medium is no longer able to amplify them. The laser medium has only so much stored energy and only so many excited systems in it. If the laser medium continues to receive additional energy, it may continue to amplify the light somewhat. If it doesn't receive more energy, light amplification will eventually cease.
To let the light out of this laser oscillator, one of its mirrors is normally semitransparent- that is, some of the photons that strike the surface of the mirror travel through it rather than reflecting. (The one-way glass used for surveillance is actually a semitransparent mirror.) This transmission creates a beam of outgoing light, a laser beam. The laser beam continues to emerge from the mirror as long as the amplification process can support it.
Because this laser beam consists of duplicates of one original photon, it is coherent light. For technical reasons, many lasers duplicate more than one original photon simultaneously, so their laser beams are a little less coherent than they might be. However, with suitable fine-tuning, one original photon can usually be made to dominate the laser beam.
When you focus a flashlight's beam with a lens, its independent photons won't end up exactly together at the focus of the lens. That's because the photons leave the flashlight heading in somewhat different directions and because their broad range of wavelengths leads to dispersion problems in the lens. However, since virtually all the photons in a laser beam are identical-they travel along the same path, and their wavelengths are the same- they can all focus together to an extremely small spot. That's why a laser printer employs a laser; a laser beam can illuminate a very small spot on the photoconductor drum that is used in the xerographic process to produce a printed image.
How a Laser Medium Works
Obtaining the excited systems needed to amplify light is a critical issue for lasers. Ideally, a laser involves four different states of an atom or atomlike system: the ground state, an excited state, the upper laser state, and the lower laser state. The reason for having four separate states should become clear in a moment.
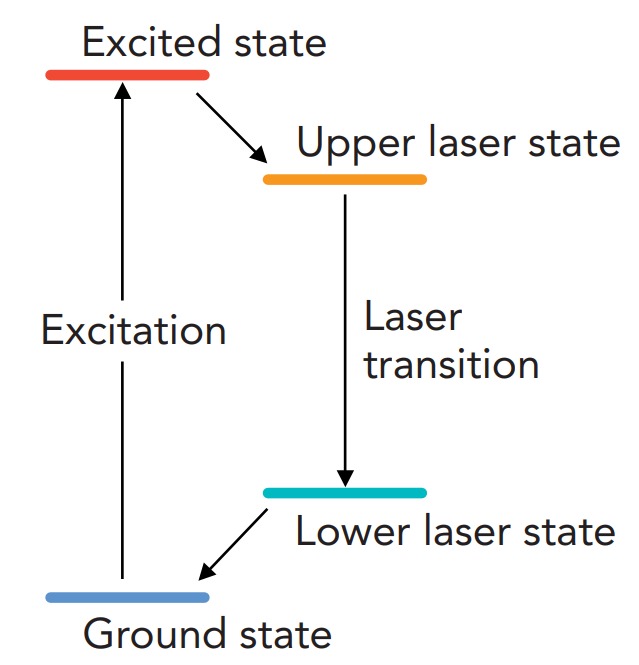
Let's consider an atom that acts as an ideal laser amplifier. The atom starts in its ground state. A collision or the absorption of a photon shifts it to the excited state, giving it the energy it needs to amplify light. The atom then shifts to the upper laser state, either by emitting a photon or as the result of a collision. This preliminary shift is important because it prevents the excited atom from returning directly to the ground state and avoiding the amplification process. Once it has shifted to the upper laser state, the atom is stuck there and will wait around long enough to amplify light.
The atom is poised to duplicate a passing photon. But not any old photon will do; it must match the photons that the atom is capable of emitting. For example, a neon atom with an excited 3p electron can duplicate a red photon but not a blue photon. This color selectivity of a laser amplifier is why most laser beams have a single, pure color.
When a suitable photon passes through the atom, that photon stimulates the emission of a duplicate photon and the atom undergoes a radiative transition to the lower laser state. So far, so good. However, if the atom remains in the lower laser state, it might absorb a photon of the laser light and return to the upper laser state. To prevent this sort of radiation trapping, the atom must quickly shift to the ground state, either by emitting a photon or as the result of another collision. The atom is then ready to begin the cycle all over again.
This four-state cycle, or something close to it, is found in nearly all lasers. The cycle helps the laser develop a population inversion between its upper and lower laser states, a situation in which there are more atoms in the upper laser state prepared to emit the laser light than there are atoms in the lower laser state prepared to absorb that light. Developing a population inversion is critical to laser amplification because, without it, the laser medium is more absorbing than amplifying and there can't be a buildup of light intensity.
In each laser, something provides the energy needed to shift atoms or atomlike systems in the laser medium from their ground states to their excited states in order to develop a population inversion. This transfer of energy into the laser medium to prepare it for amplifying light is called pumping. How a particular laser medium is pumped depends on the laser.
The most common pumping mechanisms are electronic and optical. In electronic pumping, currents of charged particles use their kinetic or electrostatic energies to excite the medium's atoms or atomlike systems from their ground states to their excited states. In optical pumping, intense light is shone on the laser medium, causing a similar excitation.
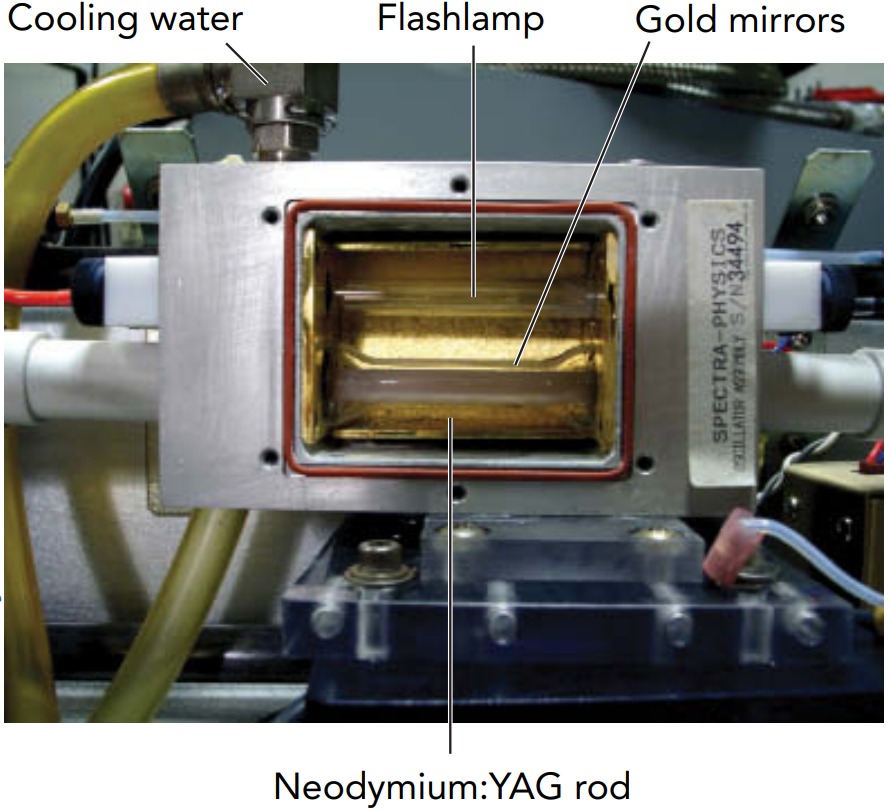
The most important examples of optical pumping are ion-doped solid-state lasers. These lasers are based on atomic ions embedded in transparent solids. Common ions are titanium (T i), neodymium (N d), and erbium (E r), and they are often embedded in sapphire, yttrium aluminum garnet (Y A G), or glass. Ti:sapphire, N d:Y A G, and E r:glass lasers are important in modern research, technology, and optical communications systems. When these laser media are exposed to extremely bright light, their ions become excited and they can act as laser oscillators or amplifiers.
A laser diode is quite similar to an LED, except that a laser diode uses its radiative transitions to amplify light. Since that amplification can occur only when light emission exceeds light absorption, the laser diode must produce a population inversion between an upper laser state and a lower laser state.
The laser diode achieves such an inversion by concentrating current into a very narrow p-n junction made from heavily doped semiconductors. The intense current injects an enormous density of electrons into the anode's conduction band, where they quickly settle into the lowest-energy conduction levels-the upper laser state. The heavy doping empties most of the anode's highest-energy valence levels-the lower laser state. With many electrons in the upper laser state and few in the lower laser state, the diode has a population inversion and can amplify light.
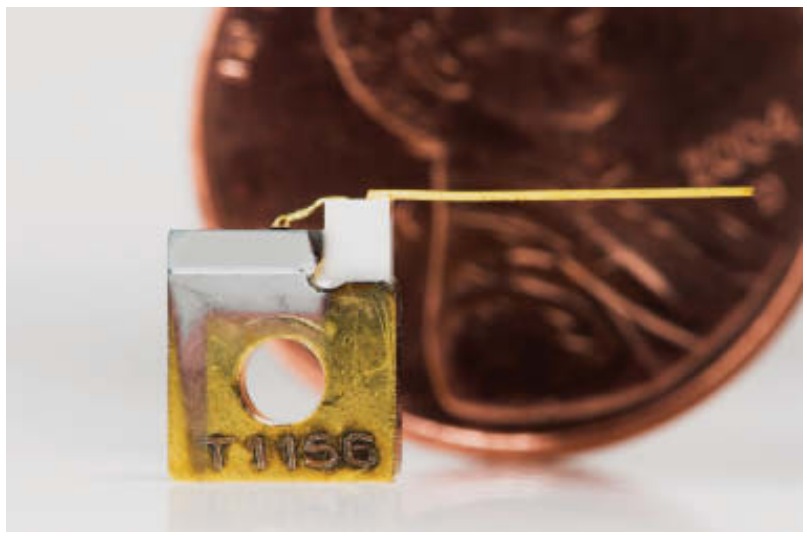
Most laser diodes act as laser oscillators, amplifying their own spontaneously emitted light until it forms an intense coherent beam. The ends of the anode itself are usually reflective enough to act as mirrors and form a complete laser oscillator. However, to concentrate the laser light in one direction and to control its beam characteristics, many laser diodes have complicated structures and coatings.
Precaution on using lasers
Laser light is dangerous. Because laser light can be extremely bright and can focus so tightly, it presents a serious eye hazard. A laser beam entering your eye will focus to a tiny spot on your retina and may cause rapid and permanent injury. Although lasers up to Class III are relatively eye-safe, they rely on your natural blink reflex to protect you. You should never stare into any laser. Class IV lasers are not eye-safe, and their light should never enter your eyes at all.